2024. 9. 20. 18:30ใScience/Biology
https://www.nature.com/articles/nature12517 (28 August 2013)
Cerebral organoids model human brain development and microcephaly
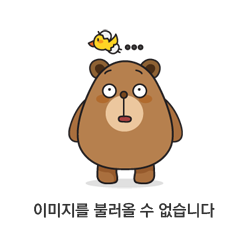
Abstract
The complexity of the human brain has made it difficult to study many brain disorders in model organisms, highlighting the need for an in vitro (์คํ ํ๊ฒฝ ์์์) model of human brain development. Here we have developed a human pluripotent stem cell-derived (์ค๊ธฐ์ธํฌ) three-dimensional organoid culture system, termed cerebral organoids, that develop various discrete, although interdependent, brain regions.
These include a cerebral cortex (๋๋ํผ์ง) containing progenitor (์ ๊ตฌ์ธํฌ) populations that organize and produce mature cortical neuron subtypes. Furthermore, cerebral organoids are shown to recapitulate features of human cortical development, namely characteristic progenitor zone organization with abundant outer radial glial stem cells(๋ฐฉ์ฌํ ์ ๊ฒฝ ์๊ต ์ค๊ธฐ์ธํฌ, RGPs).
Finally, we use RNA interference and patient-specific induced pluripotent stem cells to model microcephaly (์๋์ฆ), a disorder that has been difficult to recapitulate in mice. We demonstrate premature neuronal differentiation in patient organoids, a defect that could help to explain the disease phenotype (ํํํ). Together, these data show that three-dimensional organoids can recapitulate development and disease even in this most complex human tissue.
* Radial Glial Stem Cells, RGPs : ๋ฐฉ์ฌํ ์๊ต ์ธํฌ
- ๋๋ ํผ์ง์ ๋ชจ๋ ๋ด๋ฐ์ ์์ฑํ๋ ์ญํ ์ ํ๋ ์ ๊ตฌ ์ธํฌ
- bipolar-shaped progenitor cells that are responsible for producing all of the neurons in the cerebral cortex.
* RNA interference : RNA ๊ฐ์ญ
- ์ธํฌ ๋ด์์ ํ์ฑํ ์ํ์ ์ ์ ์์ ๊ทธ๋ ์ง ์์ ์ ์ ์๋ฅผ ๊ตฌ๋ณํ๋ ์ญํ
Discussion
We have established a novel approach to studying human neurodevelopmental processes through in vitro culture of cerebral organoids from human pluripotent stem cells (๋ง๋ฅ ์ค๊ธฐ์ธํฌ, PSCs). This method recapitulates not only fundamental mechanisms of mammalian (ํฌ์ ๋ฅ์) neurodevelopment, but also displays characteristics of human brain development. We are hopeful that this method will allow for the study of a variety of neurodevelopmental processes specific to human brain development.
Furthermore, a primary goal in neuroscience is to understand the roots of human neurological disease. We have modelled at least some aspects of microcephaly in these cerebral organoids. The finding that progenitor zones (์ ๊ฒฝ์ ๊ตฌ์ธํฌ) in patient-derived tissues display premature neural differentiation at the expense of early progenitors supports a model in which the founder population of RG progenitors fails to properly expand in patient tissues, thereby leading to an overall smaller brain. -> ์๋์ฆ์ ๋ฐํ
This may also explain why mouse models have been unable to recapitulate the severity of the disorder in humans. It is proposed that the mouse founder population of neural progenitors do not undergo expansion to the same extent as in humans before the onset of neurogenesis7. Thus, a disruption of the founder population in mice would not lead to as severe an effect as that seen in humans.
Overall, our findings suggest that we can use this in vitro culture system to model aspects of human neurodevelopment and neurological disease and hopefully provide novel insight into the pathogenesis of these disorders.
* progenitor zones - ๊ธฐ๋ฅ์ ๋ํด ์์๋ณด๊ธฐ
* RG progenitors -> ์ธ๊ฐ๊ณผ ์ฅ์ ์ฐจ์ด์ ์ ๋ํด ๋ ์์๋ณด๊ธฐ (์๋์ฆ ๋ฐํ ๊ณผ์ ์์)
Introduction
Mammalian brain development begins with the expansion of the neuroepithelium (์ ๊ฒฝ์ํผ) to generate radial glial stem cells (RGs - ๋ฐฉ์ฌํ ์ ๊ฒฝ์๊ต ์ค๊ธฐ์ธํฌ)1. These RGs divide at the apical (์ ์ ์) surface within the ventricular zone (VZ, ์ฌ์ค๋) to generate neurons and intermediate progenitors (์ ๊ตฌ์ธํฌ). Intermediate progenitors populate the adjacent subventricular zone (SVZ, ์ธ์ ํ ๋์คํ๋), whereas neurons migrate through the intermediate zone to populate specific layers within the cortical plate (ํผ์งํ).
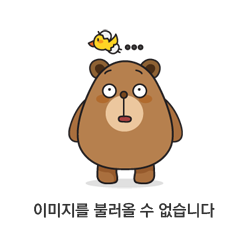
* progenitor cell : ์ ๊ตฌ์ธํฌ -> ์ ์ดํด X
- ํฌ์ ๋ฅ์ ์ธํฌ๋ ์ฒด์ธํฌ, ์์์ธํฌ, ์ค๊ธฐ์ธํฌ 3๊ฐ์ง (์ฑ์ธ์ ์ฝ 100์กฐ๊ฐ์ ์ธํฌ๋ก ๊ตฌ์ฑ)
- ์ฒด์ธํฌ - ์ผ์์ฒด ์์ ๊ฐ์ง๋ ์ด๋ฐฐ์ฒด - ํผ๋ถ์ ๊ทผ์ก ๋ฑ ๋๋ถ๋ถ์ ์ธ์ฒด ๊ตฌ์ฑ / ์์์ธํฌ - germ line cell์ ๋ฐ๋ผ ์๊ฒจ๋จ
- ์ค๊ธฐ์ธํฌ - ํนํ๋ ์ด๋ ํ ์ธํฌ๋ก๋ ๋ถํ ๊ฐ๋ฅ
- ๋ง๋ฅ์ค๊ธฐ์ธํฌ๋ ๋ค๋ฅํ(multipotent) ์ ๊ตฌ์ฒด ์ธํฌ(progenitor cell)๋ก ๋ถํ
- ์ ๊ตฌ์ฒด ์ธํฌ๋ ์ค์ ์กฐ์ง์์ ๊ธฐ๋ฅํ๋ ์ธํฌ๋ก ๋ถํ
ex) ๊ณจ์์ ์กฐํ๋ชจ ์ค๊ธฐ์ธํฌ๋ ์ฑ์ฒด ์ค๊ธฐ์ธํฌ๋ก, ์ ํ๊ตฌ, ๋ฐฑํ๊ตฌ, ํ์ํ์ผ๋ก ๋ถํ
- ๊ณจ์์ ์ค๊ฐ์ฝ ์ค๊ธฐ์ธํฌ๋ ์ฑ์ฒด ์ค๊ธฐ์ธํฌ๋ก ๊ธฐ์ง ์ธํฌ, ์ง๋ฐฉ ์ธํฌ, ๋ผ ์ธํฌ๊ฐ ๋จ
- ์ํผ ์ค๊ธฐ์ธํฌ๋ ์ ๊ตฌ์ฒด ์ธํฌ๋ก ํผ๋ถ ์ธํฌ๋ค๋ก ๋ถํ / ๊ทผ์ก ์์ฑ ์ธํฌ๋ ์ ๊ตฌ์ฒด ์ธํฌ๋ก ๊ทผ์ก ์กฐ์ง์ผ๋ก ๋ถํ
In humans, the organization of progenitor zones is markedly more elaborate; the SVZ is split by an inner fibre layer (IFL) into an inner SVZ and an outer SVZ (OSVZ)2. The OSVZ represents an entirely separate progenitor layer populated by intermediate progenitors and a unique stem cell subset termed outer radial glia (oRG)3,4, which are only present to a limited degree in rodents5. Both the IFL and OSVZ are completely absent in mice6. These key differences allow for the striking expansion in neuronal output and brain size seen in humans7,8.
Primary microcephaly is a neurodevelopmental disorder in which brain size is markedly reduced9. (์๋์ฆ์ ์ ์) Autosomal-recessive mutations (์์ผ์์ฒด ์ด์ฑ ๋์ฐ๋ณ์ด) have been identified in several genes, all of which encode proteins localizing to the mitotic spindle apparatus (์ฒด์ธํฌ ๋ถ์ด ๋ฐฉ์ถ์ฒด). Heretofore ์ง๊ธ๊น์ง, primary microcephaly pathogenesis (๋ณ์ธ, ์ง๋ณ์ด ๋ฐ์ํ๋ ๊ณผ์ ) has primarily been examined in mouse models. However, mouse mutants for several of the known genes11โ14 have failed to recapitulate the severely reduced brain size seen in human patients. (์ฅ๋ก๋ ์ธ๊ฐ์ ์๋์ฆ์ ์ค๋ช ํ ์ X, ์ฐจ์ด๊ฐ ํฌ๋ค.)
Given the dramatic differences between mice and humans, methods that recapitulate paradigms of human brain development in vitro have enormous potential. Although considerable progress has been made for in vitro models of whole-organ development for other systems, a three-dimensional culture model of the developing brain as a whole has not been established. Previous studies have modelled certain isolated neural tissues in vitro19โ23, including dorsal cerebral cortical tissue, which could recapitulate many aspects of early development.
However, later events such as the formation of discrete cortical layers with stereotypical inside-out organization as well as human characteristics, such as the presence of oRG cells and the unique organization of progenitor zones, were not described. Here we describe a three-dimensional culture system for deriving brain tissue in vitro. These so-called cerebral organoids develop a variety of regional identities organized as discrete domains capable of influencing one another. Furthermore, cerebral cortical regions display an organization similar to the developing human brain at early stages, as well as the presence of a considerable oRG (outer radial glia) population. Moreover, cerebral cortical neurons mature to form various pyramidal identities with modest spatial separation. Finally, we use patient-derived induced pluripotent stem (iPS) cells and short hairpin RNA (shRNA) in these organoids to model CDK5RAP2-dependent pathogenesis of microcephaly, a disorder that has been difficult to model in mice.
Generation of cerebral organoids
Recent progress with in vitro models of various organ systems has demonstrated the enormous self-organizing capacity for pluripotent stem cells to form whole tissues. We built upon this concept and developed a protocol without the use of patterning growth factors, focusing instead on improving growth conditions and providing the environment necessary for intrinsic cues to influence development.
We began with a modified approach to generate neuroectoderm (์ธ๋ฐฐ์ฝ) from embryoid bodies(๋ฐฐ์์ฒด). Neuroectodermal tissues were then maintained in three-dimensional culture and embedded in droplets of Matrigel to provide a scaffold for more complex tissue growth. These Matrigel droplets were then transferred to a spinning bioreactor to enhance nutrient absorption (Fig. 1a). This method led to rapid development of brain tissues, which we termed cerebral organoids, requiring only 8โ10 days for the appearance of neural identity and 20โ30 days for defined brain regions to form. -> ๋น ๋ฅด๊ฒ ์ค๊ฐ๋ ธ์ด๋๋ฅผ ์ฑ์ฅ์ํฌ ์ ์์
Cerebral organoids at early stages (15โ20 days) formed large, continuous neuroepithelia(์ ๊ฒฝ์๊ต์ธํฌ) surrounding a fluid-filled cavity reminiscent of a ventricle (๋์ค) with characteristic apical localization of the neural specific N-cadherin (Fig. 1b). Furthermore, the neuroepithelium was larger and more continuous than tissues generated using a stationary approach, which instead formed an aggregate of several small rosette-like neuroepithelia.
Although tissues reached maximal size by 2 months, cerebral organoids formed large (up to 4 mm in diameter), complex heterogeneous tissues, which could survive indefinitely (currently up to 10 months) when maintained in a spinning bioreactor. Histological and gross morphological analysis revealed regions reminiscent of cerebral cortex, choroid plexus(๋งฅ๋ฝ ์ผ๊ธฐ, ๋์ฒ์์ก ์์ฑ), retina and meninges(๋์ฒ์๋ง) (Fig. 1c, d and Extended Data Fig. 1b).
Notably, tissues typically reached a size limit, probably because of the lack of a circulatory system and limitations in oxygen and nutrient exchange. Consistent with this, extensive cell death was visible in the core of these tissues (Extended Data Fig. 1c), whereas the various brain regions developed along the exterior. Furthermore, cerebral organoids could be reproducibly generated with similar overall morphology and complexity from both human embryonic stem (ES) cells and iPS cells (Extended Data Fig. 1d, e).
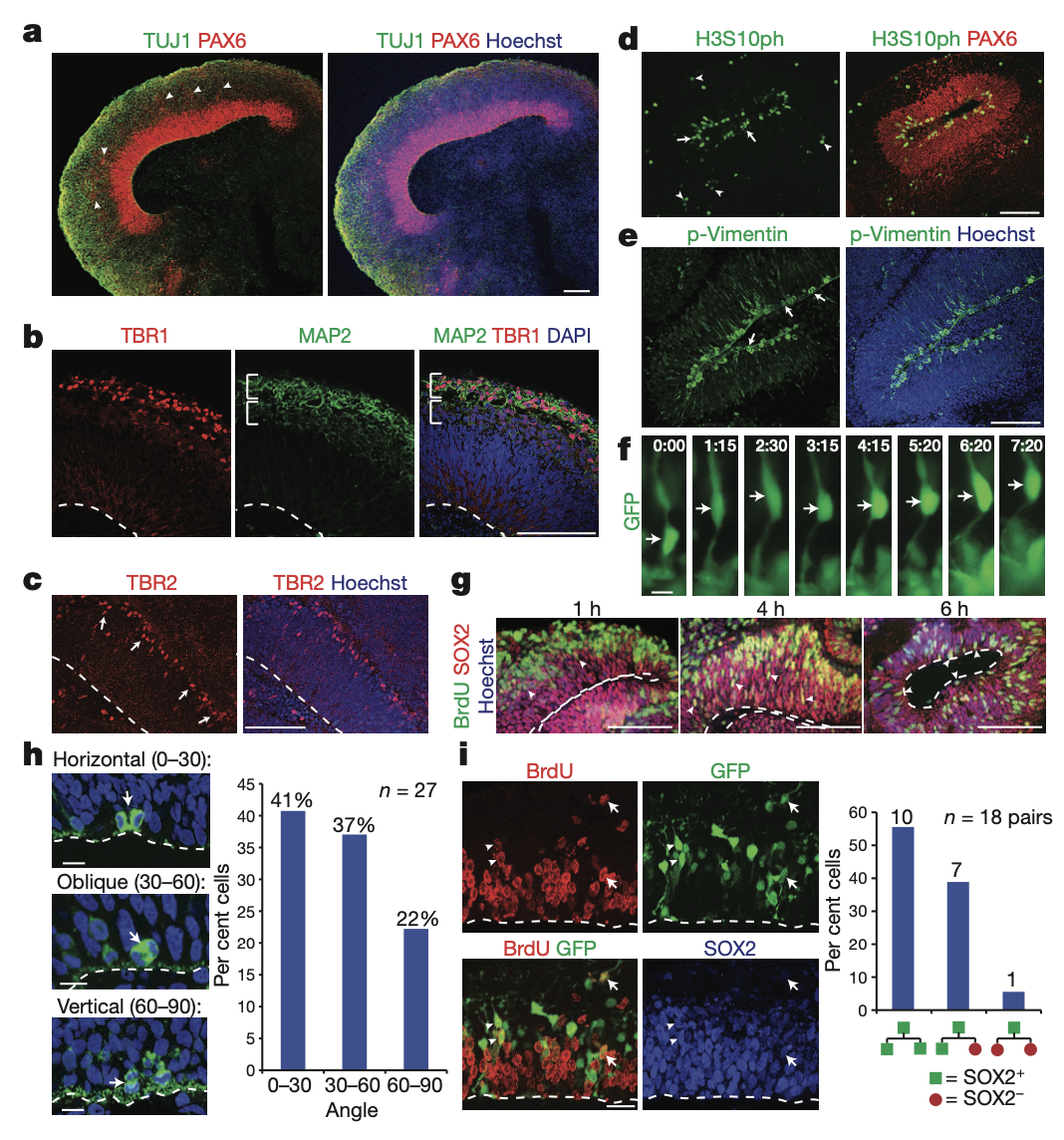
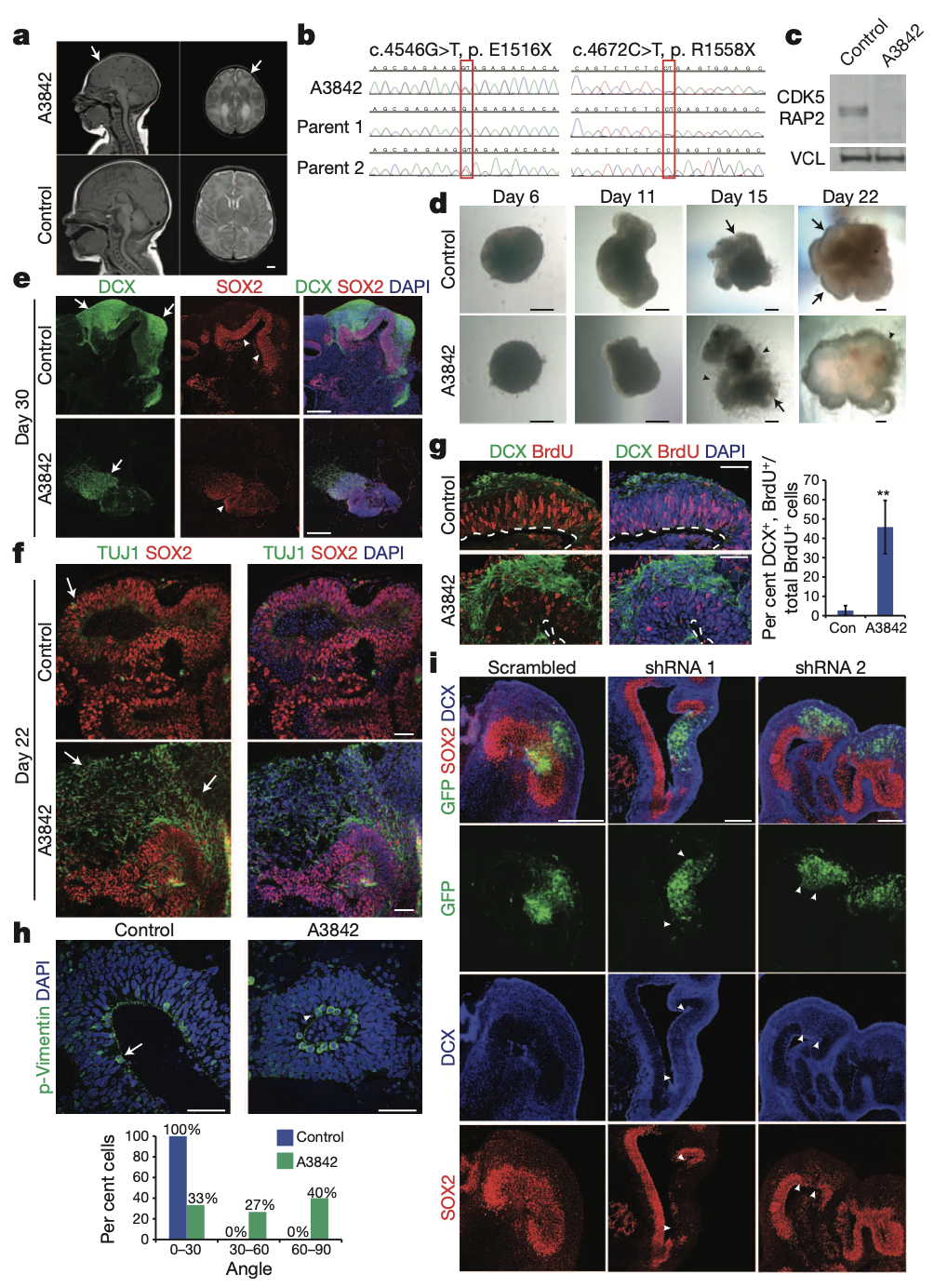