2024. 10. 23. 22:20ㆍScience/Biology
Mechanically programming anisotropy in engineered muscle with actuating extracellular matrices
THE BIGGER PICTURE
Cells within tissues communicate with each other, and with their surrounding matrix, thorough biochemical, electrical, and mechanical signals. While there are a range of techniques for mapping and controlling electrical and biochemical communication within multicellular systems, there is a significant need for tools that measure and modulate mechanical stimuli in a similar manner. A platform for dynamically patterning forces within tissues would enable mechanically programming morphology and function of various adaptive mechanobiological processes.
SUMMARY
The hierarchical design and adaptive functionalities of biological tissues are driven by dynamic biochemical, electrical, and mechanical signaling between cells and their extracellular matrices. While existing tools enable monitoring and controlling biochemical and electrical signaling in multicellular systems, (기존 방식들 - 다세포 시스템 모니터링 · 컨트롤은 가능) there is a significant need for techniques that enable mapping and modulating intercellular mechanical signaling. -> 세포 간 신호를 컨트롤
We have developed a magnetically actuated extracellular matrix that serves as a mechanically active substrate for cells and can program morphological and functional anisotropy in tissues such as skeletal muscle. This method improves the ease and efficiency of programming muscle force directionality and synchronicity for applications ranging from medicine to robotics.
Additionally, we present an open-source computational framework enabling quantitative analyses of muscle contractility. (깃허브 링크 있음) Our actuating matrices and accompanying tools are broadly applicable across cell types and hydrogel chemistries, and they can drive fundamental studies in mechanobiology as well as translational applications of engineered tissues in medicine and machines.
Introduction
Multicellularity is the key to the dynamic functionality of biological tissues. Cells embedded in an extracellular matrix (ECM - 세포외기질) communicate with each other, and with their surrounding scaffold, through a combination of chemical, electrical, and mechanical signaling. While scientists have developed a range of tools for mapping and modulating electrical and chemical signaling within tissues, including microinvasive electrodes and nanofluidic probes, there remains a significant need for robust tools that can actively tune intercellular mechanical signaling. Techniques for mechanical measurement such as traction force microscopy and stretchable biosensors can offer insight into the forces generated by living cells, but they are designed for passive observation rather than for active modulation of mechanical signaling. (기존 방법론들의 한계점)
Methods for actively imposing forces on multicellular systems have largely focused on actuated stretching and compression devices for whole-tissue stimulation, which do not replicate the spatial modulation of forces observed in natural systems. (자연계의 모방에 한계를 보임) A soft actuating substrate capable of controlling cell- cell mechanical interaction with high spatiotemporal precision could be used to probe the impact of external forces on intercellular communication and to measure downstream impact on morphology and function. Such a material would be an impactful enabling technology for engineering tissues from a range of cell types known to be impacted by mechanical signaling.
Several recent studies have reported active hydrogels controlled by external stimuli such as light, heat, pH, magnetic, and chemical inputs. Optically and magnetically responsive hydrogels have, in particular, proven highly compelling as cell substrates as they are spatially controllable and non-contact, thereby limiting off-target effects on cells. However, most prior studies on actuating hydrogels as cell substrates have focused on dynamic stiffness modulation or 3D shape transformation, rather than on the in-plane actuation that more closely mimics cell-cell contact. Moreover, they rely on synthetic polymer chemistries and cannot be readily adapted to the wide variety of natural hydrogels (collagen, fibrin, gelatin, Matrigel, etc.) most commonly used as extracellular matrix mimics. While rare reports of magnetically actuated natural hydrogels exist, their ability to generate forces across the full biologically relevant range (from pN to mN), as well as their functional impact on any specific biological tissue, has not yet been assessed, to our knowledge.
We have developed a magnetic extracellular matrix and custom-built actuation platform that can generate modular, programmable, and tunable deformation within multicellular systems. We test this platform as an active substrate for skeletal muscle, an important tissue that is responsible for all voluntary motion in our bodies and is highly adaptive to mechanical signaling.
Our study shows that we can control the directional fusion of myoblasts into aligned fibers through dynamic mechanical stimulation. Furthermore, we develop robust computational tools for tracking substrate deformation in response to muscle contraction. Our computational analysis clearly demonstrates that mechanically programmed anisotropy in muscle coordinates tissue-wide synchrony of contractile twitch. Precisely controlling muscle fiber alignment and synchronous contraction is of significant importance in tissue engineering applications ranging from medicine to robotics.
These results indicate that our technology can be used to improve the ease and efficiency of established methods to pattern muscle morphology. Beyond muscle, this study sets the stage for deploying actuating extracellular matrices as devices to map and modulate mechanical signaling within a range of multicellular tissues, enabling user-defined control of adaptive mechanobiological processes.
RESULTS
1) Design and fabrication of magnetically actuated extracellular matrices
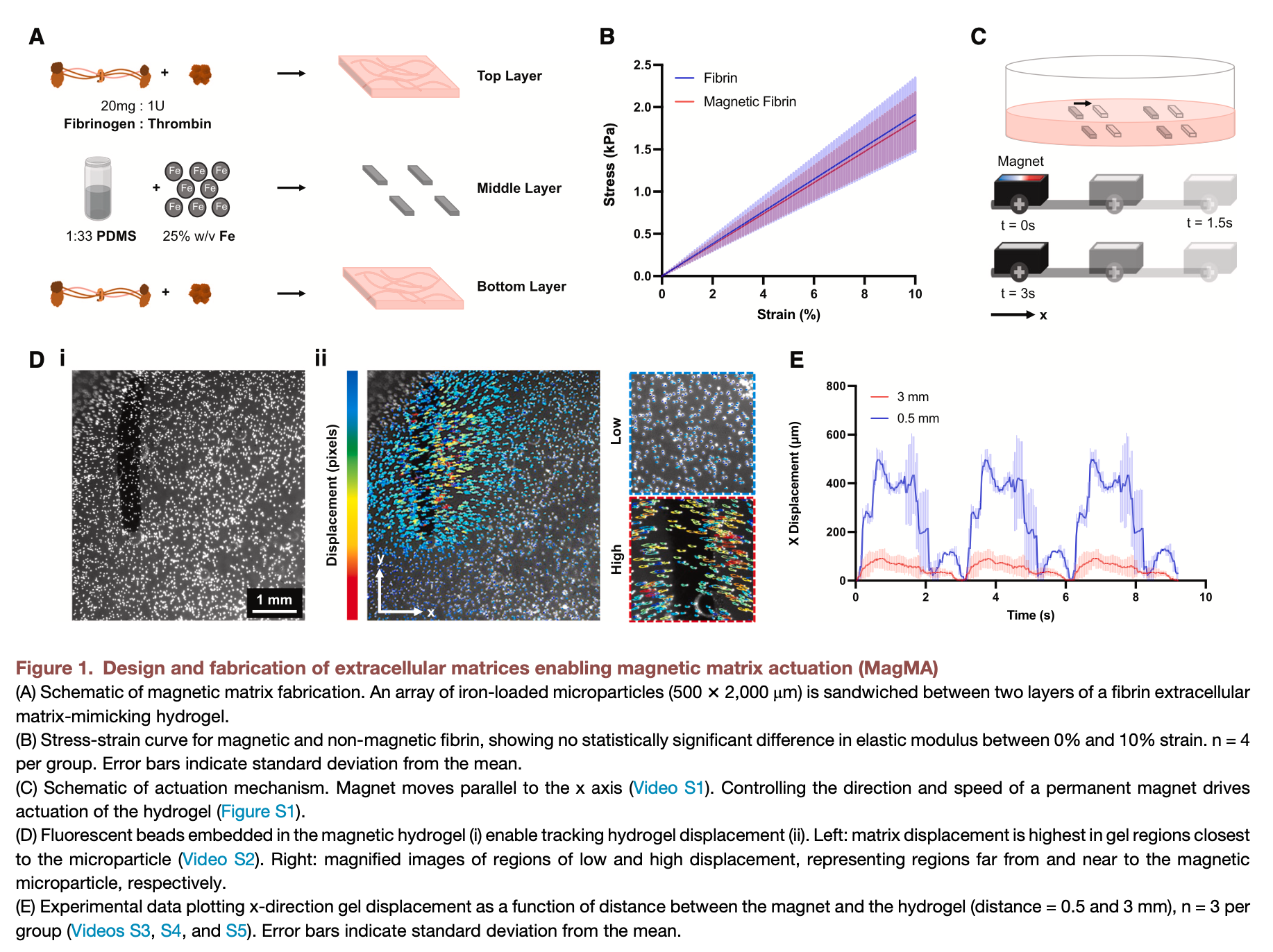
Magnetically controlled soft actuators, composed of iron nano (자기장이 working) and microparticles embedded within flexible materials, have been of significant interest in soft robotics in recent years, as they enable non-contact control of actuation with high spatio-temporal resolution.
Inspired by this approach, we mixed iron microparticles with poly(dimethyl siloxane) (PDMS) to form a sheet of magnetic silicone. We then formed magnetic silicone microparticles from this sheet and sandwiched these microparticles between two layers of a fibrin hydrogel in a cell culture plate (Figure 1A).
Compression testing of the hydrogels revealed an average elastic modulus (탄성 계수) of 18.4 ± 3.4 kPa for magnetic fibrin and of 19.1 ± 4.4 kPa for non-magnetic fibrin, confirming that there was no significant difference between groups (Figure 1B). These results indicated that the mechanical properties of the magnetic fibrin were likely suitable for cell culture. Future studies that explore the rheological properties of magnetic fibrin could further clarify whether adhered cells sense significant stiffness differences between magnetic and non-magnetic fibrin.
To dynamically control the movement of the resultant composite magnetic extracellular matrix, we constructed a custom linear actuator to move a permanent magnet under the plate with precisely controllable speed. Fluorescent beads (형광 구슬) embedded within the hydrogel were used to track matrix deformation in response to the magnetically guided movement of a microparticle, showing that deformation of the hydrogel was highest in the region closest to the microparticle (Figure 1D; Video S2). We thus embedded an array of magnetic microparticles within the fibrin hydrogels and optimized microparticle spacing to generate a more uniform deformation gradient in the region between adjacent microparticles (Figure S1A).
We tested the impact of a variety of magnetic stimulation parameters on the platform to showcase the versatility (다재) of this system. For example, we observed that for a fixed value of permanent magnet strength and microparticle composition and size, hydrogel deformation is dependent on the distance between the magnet and the hydrogel. We also tested the impact of magnet speed and orientation on the deformation of the hydrogel, demonstrating that reducing the speed of the magnet or rotating the magnet from 0 to 45 or 90 shifted the rate (speed) and direction (rotating) of microparticle movement, respectively.
These analyses demonstrated the ability of our device to enable precisely controllable magnetic matrix actuation (MagMA), motivating studying the impact of dynamic mechanical stimulation on biological tissues. As a proof-of-concept demonstration, we chose to test the impact of our MagMA hydrogel platform on muscle, a highly mechanically adaptive tissue that controls all voluntary movement in our bodies.
2) Effect of dynamic mechanical stimulation on myoblast proliferation, fusion, and maturation (근아세포 증식)
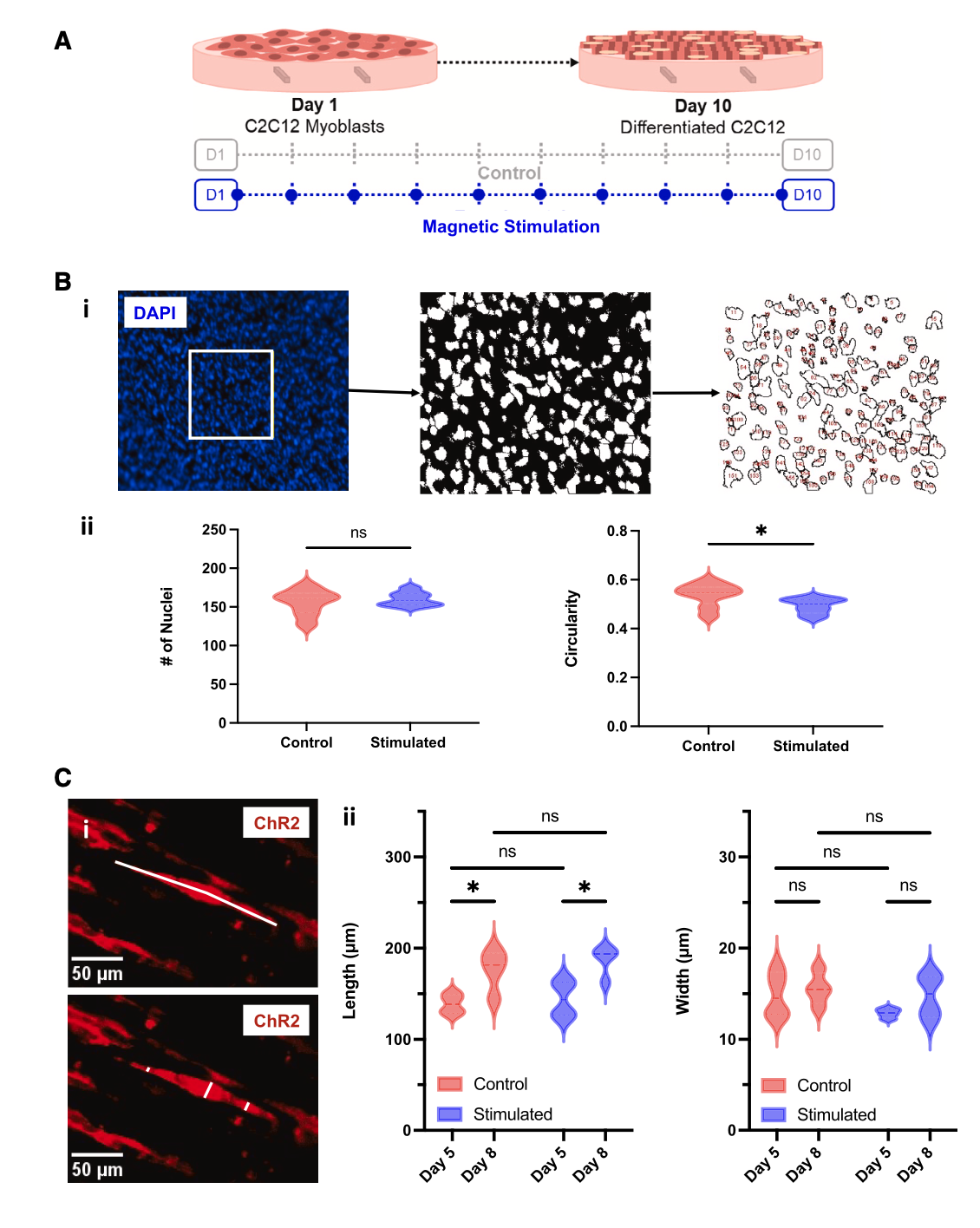
Optogenetic C2C12 mouse myoblasts were seeded on MagMA hydrogels and were maintained in a growth medium until they generated a confluent monolayer of cells after 24 h. (세포의 유입성 단층) They were transitioned to a differentiation medium after 48 h (day 0).
Our previous studies have shown that exercise training (low-frequency stimulation with 470 nm light for 30 min/day over 10 days) can dramatically improve muscle force by ~3X. (자극이 근력을 향상시킬 수 있다) We thus implemented a similar dynamic mechanical stimulation protocol to test whether mechanical training alone could similarly modulate muscle maturation (Figure 2A). We chose magnetic stimulation parameters that generated deformations similar to those generated by contractile (수축성) C2C12-derived muscle during exercise (~1%–4% strain; Figure 1E), as reported in previously published studies.42,44,45
We fixed and stained muscle tissues with a cell nuclei marker (DAPI) to assess the impact of dynamic mechanical stimulation on myoblast proliferation and fusion into multinucleated fibers. We observed no significant difference between the total number of nuclei in control and stimulated groups, indicating that our dynamic mechanical stimulation protocol had no impact on cell proliferation.
We also followed established methods to perform an analysis of circularity,45–47 as near-spherical cells (circularity ~ 1) would indicate no fusion has occurred and reduced circularity would indicate nuclei have fused and elongated. We noted that substrate actuation (기판 작동) did significantly reduce circularity, indicating enhanced fusion of myoblasts into multinucleated fibers(Figure 2B). Further morphological analysis revealed that both control and stimulated fibers grow significantly in length over time, but no significant difference in fiber length or width was observed between experimental groups on day 5 or 8(Figure 2C). -> 큰 차이가 없었다
Dynamic mechanical programming of muscle fiber alignment
Global fiber alignment is critical to native muscle architecture, as tissue-wide actuation can only be achieved by independent fibers twitching simultaneously and in the same direction. (독립적인 근섬유들이 동시에 같은 방향으로 동작) In the context of regenerative medicine, manufacturing engineered muscle that matches the form of native tissue is critical to in vivo implantation and integration. Likewise, for applications in high-throughput drug testing, quantitatively comparing the contractile force generated by muscle in physiological and pathological states requires reproducible fabrication of highly aligned constructs. Programming muscle anisotropy is perhaps most relevant in applications of muscle as a functional actuator in robots, (근육의 이방성 컨트롤이 중요한 이유) where force output and directionality must be tightly controlled to precisely regulate device funcion. There is thus a significant need, across diverse application areas, for methods to efficiently and effectively program alignment within muscle.
Existing techniques to align muscle fibers control the fusion of neighboring myoblasts through microscale geometric and biochemical cues, chronic passive stretch, and even acoustically guided cell positioning. Microscale patterning and acoustic positioning // (acoustic 의미가 무엇인지 잘 모르겠다.) require complex fabrication techniques that must be modified for each desired alignment pattern and can only be deployed at the time of tissue assembly.
Passive stretch, likewise, is chronically present throughout culture and generates non-uniform deformation gradients that cannot be spatially modulated within the tissue. There is thus a significant need for a technique enabling dynamically spatiotemporal control of the orientation of muscle fibers within engineered tissues. Our MagMA platform allows changing the rate, intensity, and angle of deformation by changing the magnetic field orientation and strength (Figures 1 and S1), providing a potential solution to this outstanding challenge in guiding muscle alignment.
-> MagMA의 장점 · 개선시킨 점
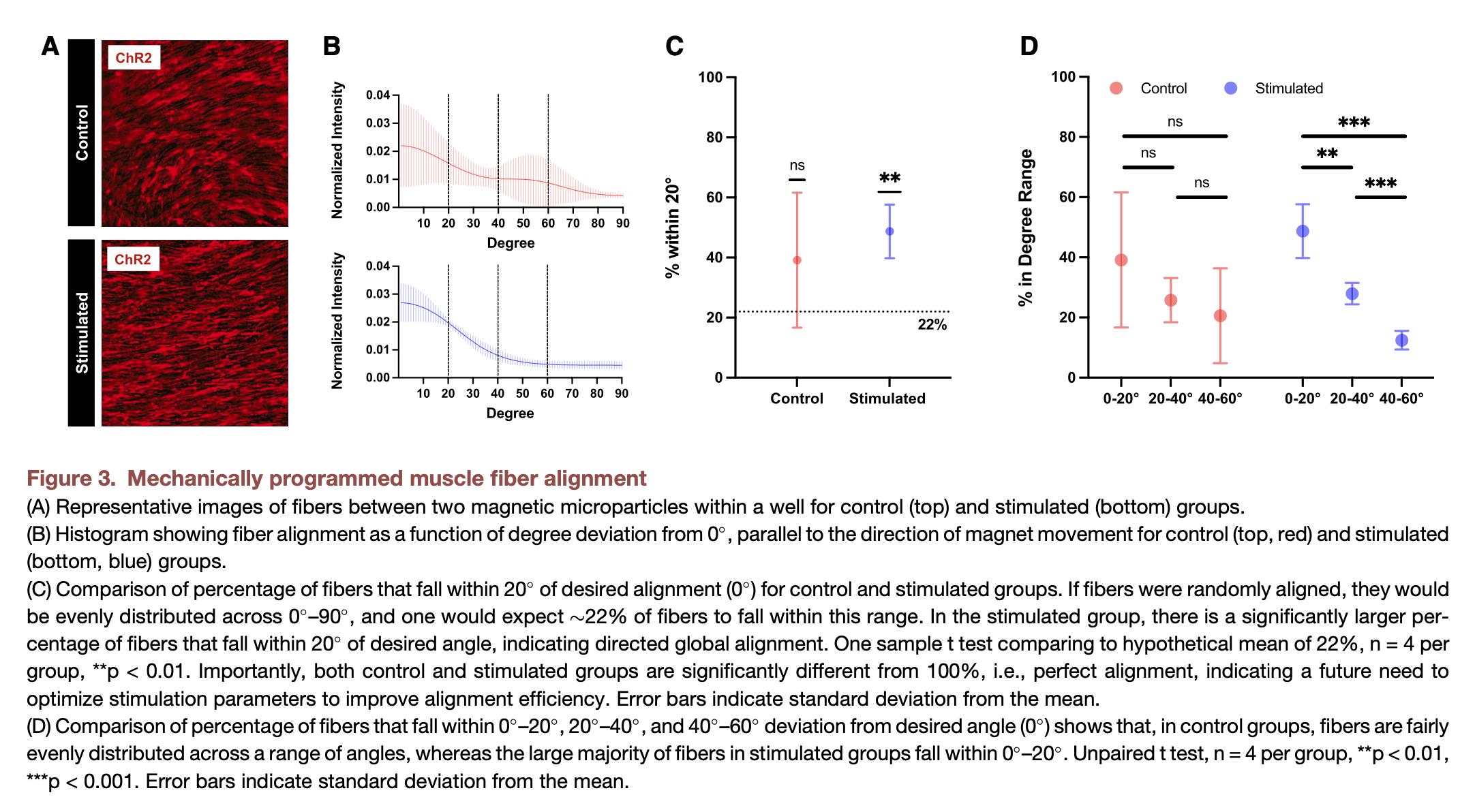
Our prior studies corroborate previous observations by others that chronic mechanical stretch (만성 기계적 스트레치) enhances fiber alignment in engineered muscle and that disrupting global alignment can have a significant negative impact on contractile force. The MagMA platform allowed us to assess, for the first time, the impact of a short period of dynamic mechanical actuation on fiber alignment. Following the stimulation regimen (섭생) outlined in Figure 2A, we fixed our muscle tissues and imaged a fluorescent marker (형광 마커) of the optogenetic membrane ion channel (ChR2 - 광유전학적 막 이온 채널).
We observed that muscle cultured on stimulated MagMA hydrogels appeared to be globally aligned, in contrast to control muscle cultured on non-stimulated MagMA hydrogels (Figure 3A - 사진에 근육들의 정렬이 잘 드러남). We used a fast Fourier transform (FFT) image analysis (이게 왜 사용되어야 하는 지 잘 이해되지 않음) technique to quantify differences in local and global alignment across control and magnetically stimulated groups. These results corroborated our visual analyses, showing that fibers in mechanically stimulated tissues were significantly more likely to align parallel to the direction of magnet movement (defined as 0 ) (Figure 3B). By contrast, fibers in control tissues did not demonstrate statistically significant global alignment and were relatively evenly distributed across a wide range of angles (Figures 3C and 3D).
Mechanically programmed alignment coordinates synchronous global contraction
We have previously shown that the contraction of optogenetic muscle can be controlled with a 470 nm light stimulus. By leveraging (활용하다) our established techniques, we optically stimulated muscle tissues on day 10 of culture and recorded videos of contraction. Quantitative comparison of motility (운동성) in complex non-homogeneous (복잡한 비균질) biological tissues is a challenging task, motivating the development of novel computational frameworks to perform this analysis in a robust, reproducible, and automated manner. We thus developed an open-source automated image processing tool to map the deformation of skeletal muscle in response to light stimulation. This tool helped us make clear quantitative comparisons between experimental groups. - 도구 개발의 필요성
* Optogenetics is a technique where a cell is transduced with a light-sensitive ion channel
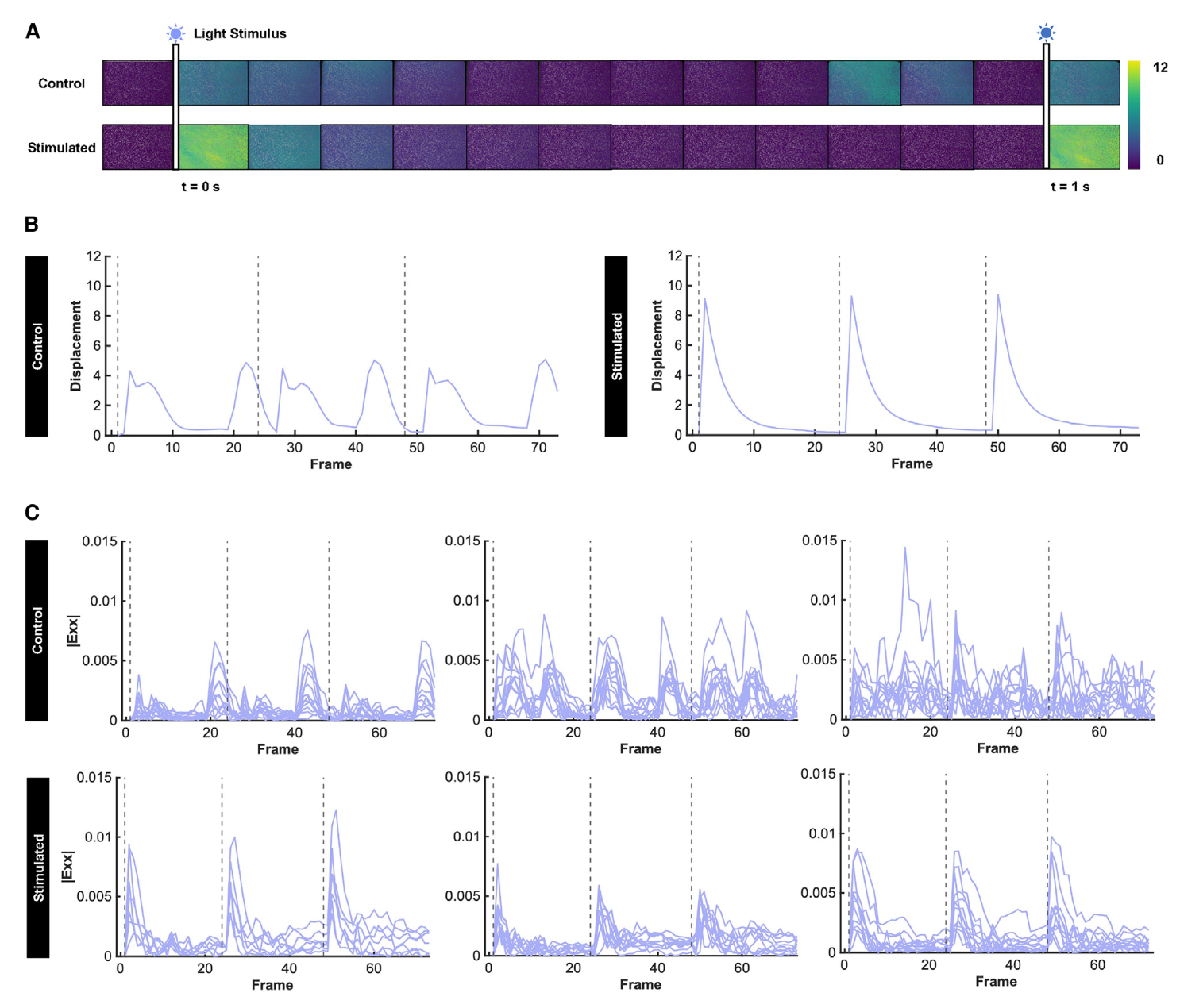
Optical stimulation of engineered muscle generated globally coordinated twitch deformation in mechanically programmed tissues, while control tissues displayed asynchronous and arrhythmic contraction (부정맥 수축) (Figures 4A–4C; Videos S6, S7, S8, and S9), indicating that improved global fiber alignment had an observable downstream impact on function.
To better visualize and quantify differences in the global synchrony of muscle contractility, we mapped deformation into normal strain along the x axis (0 , parallel to magnet movement) and visualized strain across multiple regions within each sample (Videos S10 and S11). Plotting the subdomain-averaged strain of selected regions within the image, including regions closer to and farther away from the magnetic microparticles, enabled tracking the direction and timing of the twitch in response to three sequential light pulses (Figure 4C).
Our analyses revealed that, across multiple samples and multiple regions within each sample, tissues mechanically programmed via MagMA hydrogels demonstrated a coordinated twitch, while control tissues were asynchronously contractile. These data indicate that in the absence of global alignment, muscle fibers deform the substrate in multiple different directions during contraction.
The amount of tissue displacement (변위) generated along a given direction is proportional to the number of fibers oriented at that angle. Non-uniform distribution of fibers (섬유들의 불균일한 분포) at various angles generates non-uniform spatial forces that seem to change the resultant relaxation (이완) properties of the tissue, thus reducing twitch synchrony. Overall, our functional assays corroborate the observation that the MagMA platform mechanically programs anisotropy of both form and function in engineered muscle.
DISCUSSION
Soft actuating substrates for cell culture are of significant interest for actively controlling mechanical signaling within tissues. In the context of skeletal muscle, the ability to simply and dynamically program fiber alignment is important for tissue engineering applications ranging from regenerative medicine to biohybrid robotics. Here, we show that MagMA-driven dynamic mechanical stimulation controls the directionality of fusion between neighboring myoblasts into mature muscle fibers, thus robustly programming global alignment and coordinated contractility.
We have observed that myoblasts cultured on soft gels will differentiate into locally aligned fibers within small regions (~100s of mm), likely because of traction forces (견인력) imposed by single cells on their neighbors. However, this passive culture technique does not program the global alignment required to generate coordinated contraction in the mm- and cm-scale tissues relevant for real-world applications. // 실제로 활용할 수 있을 정도로 큰 scale에서의 control이 어려웠다.
Several prior studies have demonstrated techniques for globally aligning muscle fibers within engineered tissues via microscale geometric cues, biochemical patterns, passive mechanical stretch, and acoustically guided cell positioning. While these techniques are effective, they can only be deployed at the time of cell seeding and are not designed to be dynamically changed or spatially controlled over time. // MagMA의 두드러지는 장점
For example, micropatterned substrates must be redesigned and manufactured for each desired alignment pattern. By contrast, the MagMA platform enables dynamically tuning stimulation parameters (such as magnet orientation angle) to change alignment patterns without altering the substrate fabrication process. Moreover, MagMA is dynamically deployable and could be used, in the future, to program fiber orientation at any point prior to terminal differentiation of cells.
Our actuating extracellular matrices allowed us to decouple (분리하다) the mechanical and biochemical effects of exercise by independently studying the effects of dynamic mechanical stimulation alone on fiber formation and maturation. // 기계적인 자극만 분리하여 연구할 수 있다. In prior studies, we and others have shown that exercise (30 min/day, 10 days) increases muscle force by ~3X and that combining exercise with chronic (만성의) mechanical stretch synergistically increased force by an additional 50%. (근력을 높이는 방안들)
These functional improvements were correlated with upregulation of key myogenic regulatory factors. (주요한 인자가 됨) Our previous studies indicate that exercise impacts the size and maturation of muscle fibers, whereas this study demonstrates that dynamic mechanical stimulation in the absence of exercise modulates myoblast fusion and muscle fiber alignment. // 운동 없이 기계적인 자극 -> 근아세포 융합 · 근섬유 정렬을 조절함
Mechanical stimulation alone is thus not equivalent to exercise, providing a potential explanation for the previously observed synergistic increases in muscle force (근력의 증가 시너지) in response to combined biochemical and mechanical stimulation. These results motivate future studies using MagMA in combination with other modes of stimulation. It is important to note that while we observed no impact of mechanical stimulation on proliferation rates, (증식 속도에는 영향으 미치지 않음) others have observed actuation-mediated modulation of myoblast proliferation (근아세포 증식) after longer stimulation periods (~5–17 h). These differing results indicate that varying the duration, magnitude, and frequency of mechanical stimulation can significantly change functional outcomes and could be used to further tune muscle mechanobiological processes beyond fiber alignment in future studies.
Interestingly, we have found that tension cues do not need to be chronically present throughout maturation, as was previously assumed by muscle researchers in both in vitro and in vivo contexts. Rather, a short daily regimen (일일 요법) of dynamic mechanical stimulation (30 min/day, 10 days) can sufficiently program long-lasting functional anisotropy in muscle.
This is an important advantage of our MagMA platform, as it allows on/off control of dynamic mechanical stimulation, allowing researchers to study the separate and combined impact of biochemical, electrical, and mechanical stimuli on multicellular systems during key periods of assembly and maturation.
Our study is a proof-of-concept demonstration (개념 증명) that modulating intercellular forces in skeletal muscle during differentiation can dynamically program tissue anisotropy. // 분화 단계에서 골격근 세포간 힘을 조절하면 -> 근섬유 조직의 이방성을 동적으로 프로그래밍할 수 있음을 보였다.
It is important to note that we have not optimized how modulating system parameters impacts muscle morphology or function. Future work to improve the efficiency and complexity of patterned alignment will leverage our platform’s flexibility in modulating the frequency, duration, directionality, and timeline of mechanical stimulation (Figures 1 and S1).
Beyond muscle, magnetically actuating hydrogels address a key need in tissue engineering by enabling researchers to modulate forces within tissues and study the impact on architecture and function. The rate, degree, and angle of gel deformation can be simply tuned by changing the size and distribution of the magnetic microparticles or the orientation of the magnet. Moreover, this platform can be readily adapted to a variety of natural and synthetic hydrogel chemistries that are well suited to a range of cell types. We anticipate that actuating extracellular matrices will be useful devices for programming microscale hierarchy in engineered muscle, and that this platform will drive a broad range of fundamental and translational studies of mechanobiology, regenerative medicine, and biohybrid robotics.